-
-
- Airfoil Camber
-
- The Wind Tunnel: A Controlled Environment
- The Pressure Connection
-
- Hydrodynamica
- Incorrect Applications of Bernoulli
- Flying the Barn Door
-
-
-
-
On a windy December morning in 1903, two brothers from Dayton, Ohio, made history. In just 12 seconds, the dream of powered human flight became a reality.
Aeronautical science has advanced dramatically since the Wright brothers’ flight at Kitty Hawk. It’s tempting to assume we’ve solved the mysteries of flight.
But did you know that scientists still debate how a wing creates lift? There is yet to be a singular explanation for the force that keeps airplanes aloft.
In this article, we’ll explore how early aviators discovered the magic of lift. We’ll also find out why, despite decades of research, engineers still can’t agree on how wings do what they do.
Key Takeaways
- Bernoulli’s theorem describes how pressure differences on a wing create lift.
- Newton’s laws of motion describe how the downward deflection of air creates an upward lift force.
- Neither Newton’s laws nor Bernoulli’s theorem entirely explain how wings generate lift.
Inventing the Wing
You might wonder how the airplane wing came about, considering we don’t fully know how lift works.
Well, the short answer is that we copied the blueprint from nature.
In the early 19th century, British inventor Sir George Cayley translated the shape of a bird’s wing into the modern airfoil. An airfoil is a shape designed to create lift.
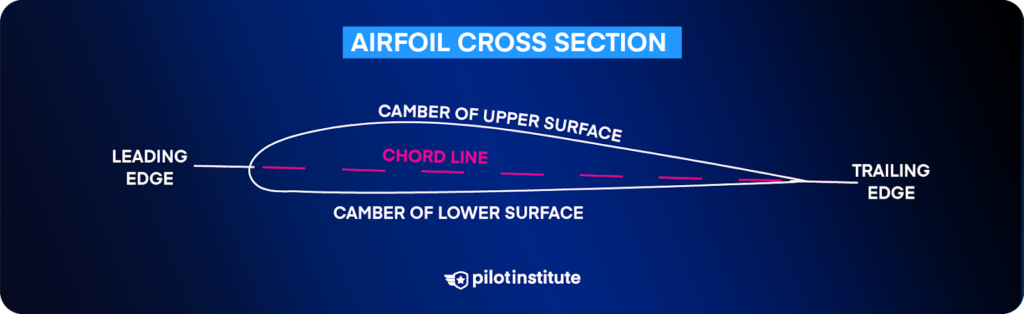
Cayley’s studies led him to discover the importance of camber. Camber is the curvature of a wing. Airfoils with a larger curve on top than the bottom have a positive camber. Symmetrical airfoils have the same curve above and below the chord line. The chord line is an imaginary line from the wing’s leading edge to its trailing edge.
Airfoil Camber
Cayley discovered that a wing with a positive camber created more lift than a symmetrical or flat wing. Cambered wings were also more resistant to stalling.
His investigations didn’t stop there. Cayley pinpointed the four forces of flight: lift, weight, thrust, and drag. He also wrote extensively on aircraft control and stability. Amazingly, Cayley did this about 100 years before the Wrights’ first powered flight.
Many of Cayley’s insights hold up surprisingly well. In his 1809 paper “On Aerial Navigation,” he suggested that the upper camber “creates a slight vacuity.” In other words, the curved top of the wing creates a partial vacuum when air passes over it. This more than hints at one of the scientific theories of lift we’ll discuss later.
The Origins of Modern Aeronautics
The invention of the wind tunnel by Frank Wenham in 1871 was a turning point in our understanding of the physics of lift. A wind tunnel is a device that allows engineers to test an airfoil’s lift, drag, and pressure distribution. Finally, inventors could consistently test their wing designs.
The Wrights would put Wenham’s invention to good use 30 years later.
The Wind Tunnel: A Controlled Environment
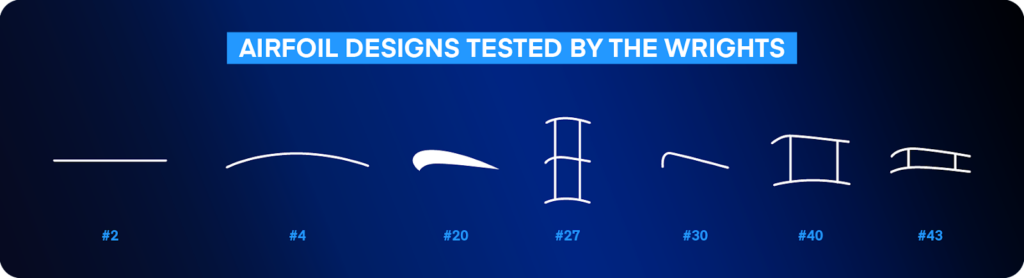
During the 1900-1901 flying season, the Wright brothers struggled with their glider design. Their wing provided less lift than data from their late friend Otto Lilienthal said it would.
So, in the autumn of 1901, the Wrights built the second wind tunnel in the United States. Their wind tunnel was the key to their successful 1903 airfoils. And they didn’t even have to leave their bicycle shop to use it.
The Pressure Connection
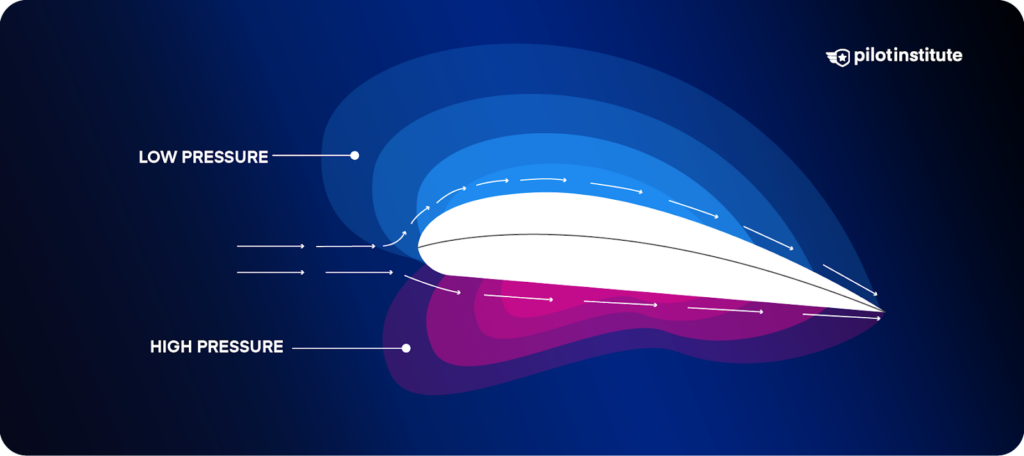
George Cayley knew back in 1809 that air pressure was lower on the top of an airfoil than on the bottom.
The Wrights clearly understood the association between accelerated airflow on the top of the wing and lower pressure. They knew that the pressure difference between the top and bottom of the wing creates lift. But the Wrights never published any theories on why this might be.
It turns out the explanation would come from an 18th-century Swiss mathematician.
Bernoulli’s Theorem
George Cayley and the Wright brothers never mentioned Daniel Bernoulli by name in their writings, but they indirectly echoed his discoveries.
Bernoulli was born in 1700 into a family of mathematicians. Unsurprisingly, he became a mathematician as well.
But at his father’s request, he also studied medicine. His exploration of blood pressure gave him insight into the physical properties of fluids.
Hydrodynamica
In his 1738 work Hydrodynamica, Bernoulli used the principle of conservation of energy to describe fluid dynamics.
Bernoulli’s theorem states that when the velocity of a fluid increases, the static pressure decreases, and vice versa. This relationship applies to any fluid in a streamline: liquid or gas.
Bernoulli never applied his theorem to the creation of lift in his lifetime. However, it explains what we see with airflow over a wing.
The air on top of the wing moves faster, corresponding with an area of low pressure. The higher pressure under the wing pushes up toward the lower pressure over the wing. This pressure differential is the lift force.
Bernoulli’s theorem became a popular way to explain lift in the following decades. However, it fails to explain why the top of the wing accelerates the air in the first place. This confusion drove many incorrect explanations over the years.
Incorrect Applications of Bernoulli
One common misunderstanding of Bernoulli’s principle is the “equal transit-time” theory.
The explanation goes something like this.
When a parcel of air hits the airfoil’s leading edge, it “splits” in two. The curved top of the airfoil creates a longer path for air molecules to travel than the bottom. So, for the air molecules on the top to meet up with air molecules on the bottom at the trailing edge, they must go faster. This increase in velocity causes a decrease in pressure, creating lift.
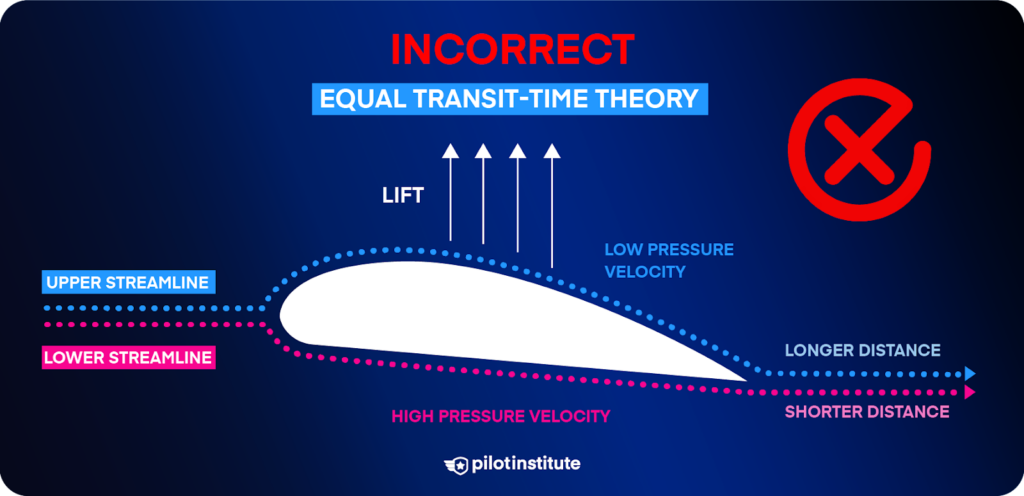
While this argument is tempting, it doesn’t stand up to scrutiny.
First, there is no reason why the air on top must simultaneously meet back up with the air on the bottom. The molecules are not “bound” to each other in any way.
Second, the air on top accelerates to a faster speed than this explanation requires. A parcel of air above the wing reaches the trailing edge before its below-wing equivalent.
As it turns out, a cambered wing is not required for lift production. Symmetrical airfoils work just fine.
Stranger still, even a flat plate generates lift.
Flying the Barn Door
There’s an old saying in aviation that goes, “with a big enough engine, you can fly a barn door.”
And as silly as it sounds, there’s some truth to that. As un-aerodynamic as a barn door is, it can produce lift.
But how?
One key aspect of lift generation is the wing’s angle of attack. The angle of attack is the angle between the chord line of the wing and the relative wind. The relative wind is the direction the wing “feels’ the air is coming from. For an airplane, the relative wind is always opposite the direction of travel.
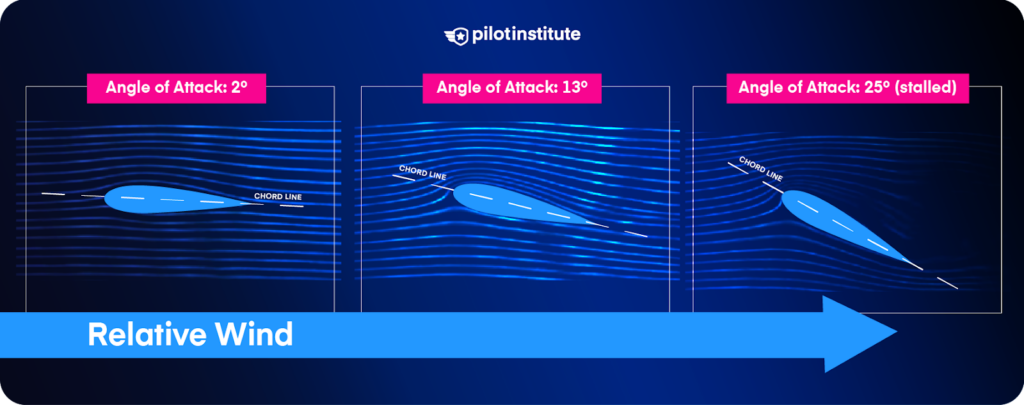
As the angle of attack increases, the pressure difference between the top and bottom of the wing increases. This differential causes lift to increase. Up to a point, anyway.
Once the wing reaches the critical angle of attack, any further increase in angle causes the airflow to separate from the wing’s surface. At this point, the wing stalls, and lift is significantly reduced.
A flat-plate wing creates extremely turbulent airflow at a higher angle of attack. The wing stalls quickly. But at a very low angle of attack, a flat wing works much like an airfoil, just not very efficiently. (A smooth shape resists stalling.) Airflow splits in two, and the top side has a lower pressure than the bottom, creating lift.
If that’s hard to believe, try it for yourself using NASA’s interactive airfoil simulation.
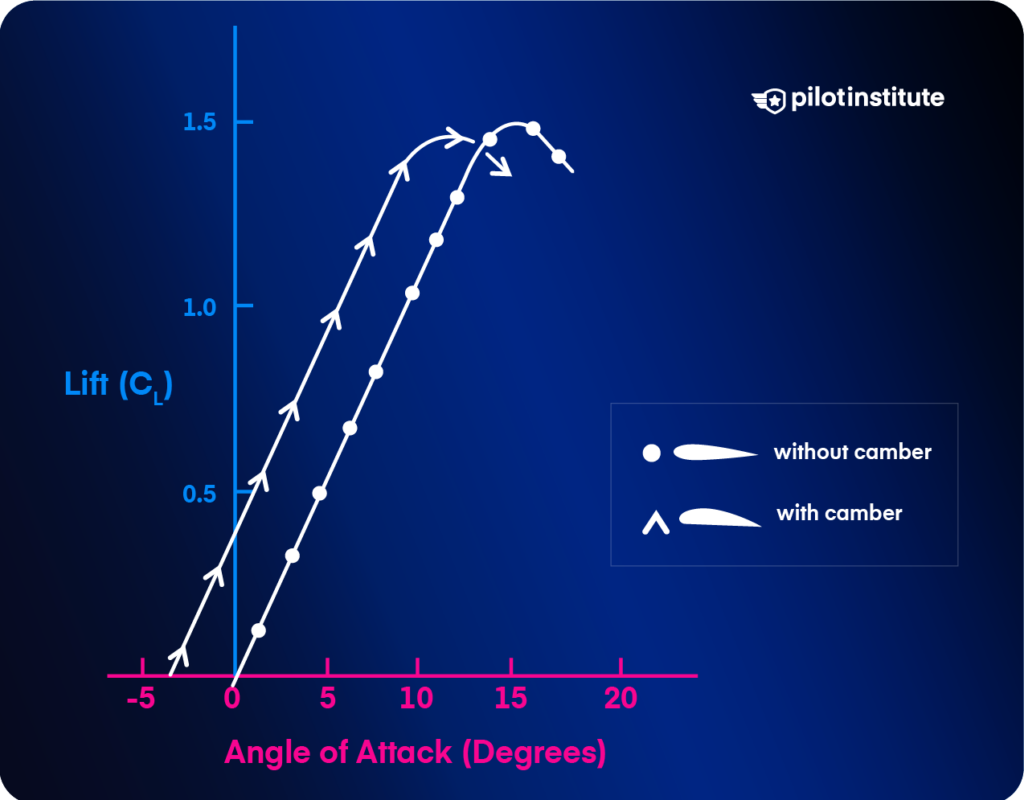
Similarly, a symmetrical airfoil works just fine to create lift. At zero angle of attack, the top and bottom pressures are equal, so there is no lift. But when the angle of attack increases, we see similar pressure patterns as with positively cambered airfoils.
Symmetrical airfoils are perfect for aerobatic airplanes that frequently fly inverted. But cambered airfoils can fly upside down as well. This feat simply requires a higher angle of attack.
So, not only is camber unnecessary to generate lift, but its lift-enhancing effects can be “overpowered” by angle of attack.
If lift doesn’t require a curved airfoil, how do we explain what creates the low pressure on top of a wing?
Enter Newton.
Newton’s Laws of Motion
Isaac Newton’s 1687 masterwork Principia took the scientific world by storm. For the first time, science could mathematically explain the physical motion of bodies.
Newton’s second law of motion states that any time a mass is accelerated, it imposes a net force. By acceleration, we mean a change in the speed or direction of the mass.
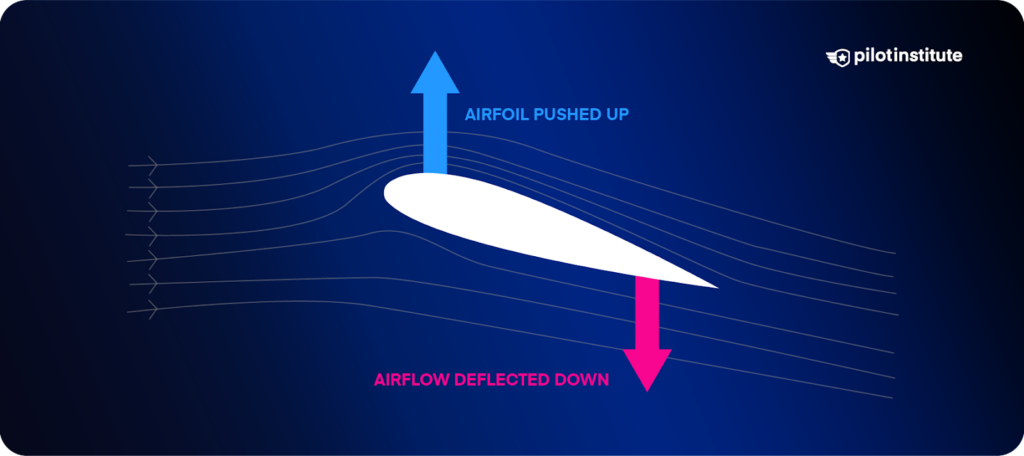
Although we don’t often think of air as particularly “heavy,” it has mass. When an airfoil slices through the air, its shape and angle of attack cause the airflow to accelerate and deflect downward. This “downwash” follows the airfoil’s contour, imparting a downward force.
And here’s where Newton’s third law comes in. This law states that for every action, there is an equal and opposite reaction. That is the conservation of momentum. So, when the airfoil accelerates the air downwards, an equal and opposite force accelerates the airfoil upwards.
This upward force is another way to explain lift.
Newton’s laws are not in opposition to Bernoulli’s theorem. They both describe the same lift force through different means. In fact, you can derive Bernoulli’s theorem directly from Newton’s second law.
Describing Lift is not Explaining Lift
You may have guessed that this is where the explanation of lift takes a complicated turn. Neither Bernoulli’s theorem nor Newton’s laws explain why an airfoil creates lift in the first place. And neither directly explains why the airfoil turns the air downward. They only describe the manifestations of lift.
We can think of lift as a relationship between four elements: an increase in airflow speed over the wing, a decrease in pressure over the wing, an increase in pressure under the wing, and a downward turning of the airflow.
The missing piece of the puzzle is what ties these elements together.
How do we create a complete theory of lift? Unfortunately, there is no easy solution to this predicament.
A Unified Theory of Lift?
Do you plan on diving deeper into the origins of lift? Be ready to come face-to-face with several complicated theories and models.
There are pressure and velocity fields around the airfoil to consider. Don’t forget the Navier-Stokes equations. And then there’s the impact of viscosity to study.
The Coandă effect seems promising to explain why air follows the airfoil’s curve. However, its direct application to the explanation of lift is controversial.
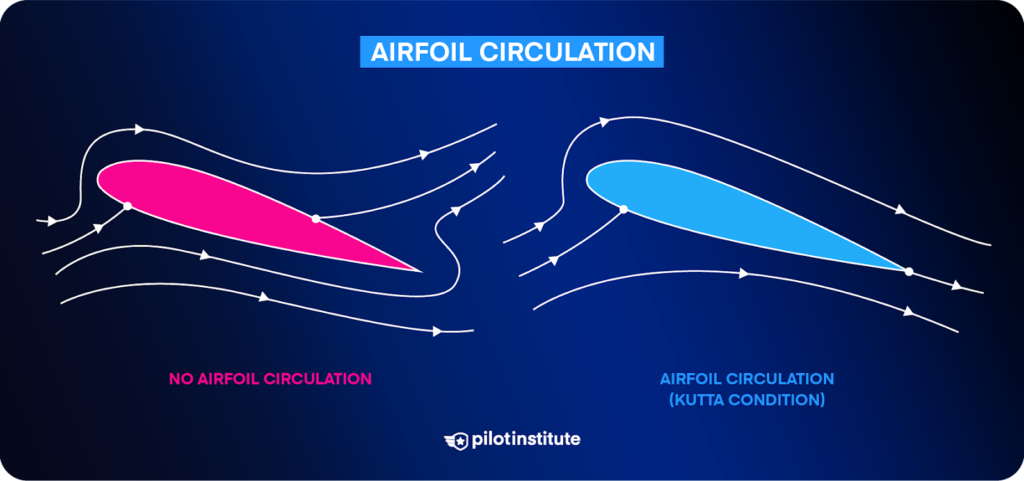
The Kutta-Joukowski theorem describes circulatory airflow around an airfoil. It’s a common explanation for how lift starts on a wing. However, it only mathematically expresses the circulation. It doesn’t provide a physical cause.
Even in 2022, scientists are still working on new theories of lift. But one singular, clear explanation of lift has yet to satisfy all the requirements.
We may be waiting quite a while for a Unified Theory of Lift.
Conclusion
Aeronautical science has come a long way since those primitive years of wooden wind tunnels and hand-plotted graphs.
Today, we can design aircraft with a level of precision early aviators only dreamed of. Modern aircraft are faster, more efficient, and safer than ever before. But even with our sophisticated computer models, we still cannot provide a universal explanation for lift.
So, be wary of those who claim to have a complete and straightforward answer. Their confidence likely outweighs their knowledge.
Want to learn more about the relationship between lift and drag? Check out the article Induced Drag Explained.