-
-
-
-
-
- Stall Awareness
- Steep Turns
- Slow Flight
- Aircraft Weight
- Stretching the Glide and Exceeding Critical AoA
-
- Wing Size and Shape
- Ice
- Wing Contamination
- Load Factor
- Aircraft Speed
- Center of Gravity
- Control Inputs
- High Lift Devices
-
When you start flying training, you’ll often hear the angle of attack referenced. But what is the angle of attack, and what is its effect? Having a good understanding of this basic flying concept is crucial. Today we will run through what angle of attack means, the factors that affect it, and some key things to keep an eye on. Let’s dive right in.
What is Angle of Attack – In Simple Terms
The angle of attack is the angle at which the oncoming airflow meets the wing. It is measured using the wing’s ‘chord line’, a straight line drawn from its leading edge to the trailing edge. The angle of attack is independent of pitch attitude, and many factors affect it.
How important is the angle of attack?
Well…
The angle of attack influences the aircraft’s behavior on a fundamental level. It is one of the main factors affecting lift, and airplanes need a constant source of lift to fly. Understanding what angle of attack is absolutely vital.
Here’s what you need to know.
How Does Angle of Attack Work?
As we’ve already stated, the angle of attack is the angle formed between the aircraft wing and the oncoming airflow.
You’ll see it written in a few ways: –
- Angle of Attack
- AoA
- Alpha
- α
Regardless of how it is written, the above all relate to the same thing. And here’s how AoA is calculated: –
When viewed in cross-section, wings are curved.
Ever tried to measure an angle from a curved surface?
Kind of tricky, right?
For this reason, aircraft theorists use something called the chord line. You can read more about chord lines here. If you want a quick recap, the chord line is a straight line intersecting the wing. It runs from the trailing edge through to the leading edge. This forms an ideal (and straight) datum allowing us to measure the angle of attack.
When an aircraft moves forward, the air produces a resultant force. This resultant force is called relative airflow.
The angle of attack is the angular difference between the relative airflow and the wing’s chord line.
Want two really simple general rules explaining how the angle of attack works?
- A greater angle between the chord line and the relative airflow means a higher angle of attack.
- A lower angle between the chord line and the relative airflow means a lower angle of attack.
Quite easy so far?
In practice, it can be a little more complex.
As we stated a little while ago, understanding the angle of attack is fundamental to understanding aerodynamics and how lift is generated.
How does it work?
Well, here’s some more detail about the angle of attack and lift.
How Does Angle of Attack Affect Lift?
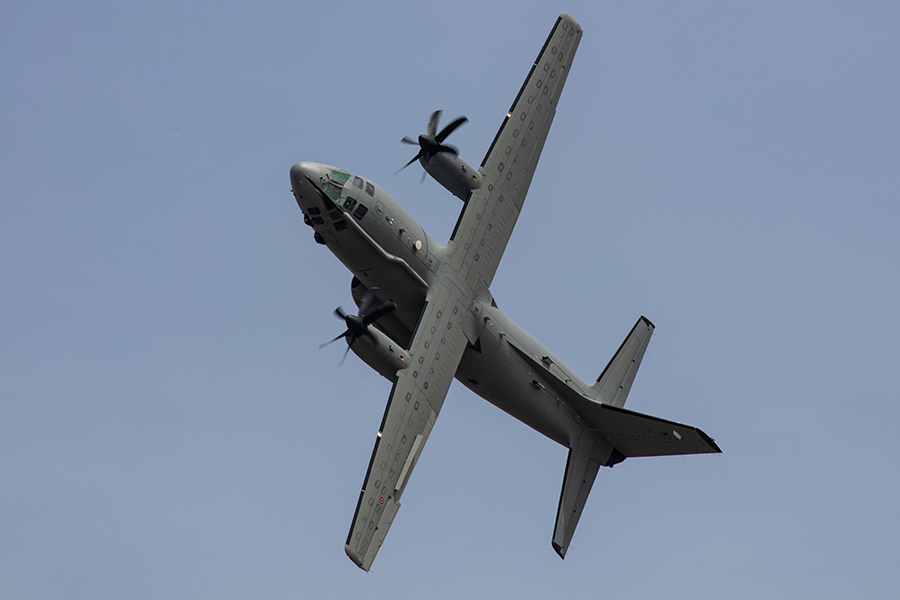
The angle of attack is directly linked to the lift. As a general rule, if everything else remains constant, the higher the angle of attack, the more lift a wing produces. Pilots maneuver the aircraft around the sky by changing the angle of attack.
Yep, it is that vital.
How does it happen?
Get your calculators ready because a simple formula can demonstrate how the angle of attack affects lift.
Let’s look at the formula for lift and see how the angle of attack fits into the bigger picture.
- L= CL x 1/2ρv2 x S
We know what you are thinking….
Jeez! That looks complicated!
Don’t worry; here at Pilot Institute, we love making difficult concepts simple, and this will be no exception.
Want the above equation in plain terms? It sounds something like this: –
The total lift produced (L) is a result of multiplying: –
- The Coefficient of Lift (CL)
- The aircraft speed through the air at a given density (1/2ρv2)
- The surface area of the wing (S)
When discussing the angle of attack, we are most concerned with the coefficient of lift (CL).
The coefficient of lift is a function of the angle of attack. Generally, the lift coefficient increases if we increase the angle of attack.
Let’s plug in arbitrary numbers to demonstrate how the angle of attack works. We’ll keep the wing shape and airspeed the same numbers. We’ll change the coefficient of lift (simulating a change in the angle of attack).
Don’t worry too much about the numbers chosen. We are just demonstrating the concept.
- Lift = CL x 1/2ρ v2 x S
- Lift = coefficient of lift x Airspeed x Wing Surface Area
- Lift = 3 x 5 x 5
- Lift = 75
In this above example, the CL had a value of 3. Remember how we said the lift coefficient is directly related to the angle of attack? If the angle of attack increases, so does the coefficient of lift. Let’s double our angle of attack, effectively increasing our lift coefficient, plug in the numbers, and see what we get…
- Lift = CL x 1/2ρv2 x S
- Lift = coefficient of lift x Airspeed x Wing Surface Area
- Lift = 6 x 5 x 5
- Lift = 150
Hopefully, the above demonstrates an important concept.
Provided all the other numbers remain constant, increasing our angle of attack increases the lift that the wing produces.
And, we can deduce another important thing from the above equation…
Suppose you reduce the value of either of the other two numbers (airspeed or wing surface area). What do you think you need to do to the angle of attack to ensure the lift remains constant?
That’s right, increase it!
That’s precisely why an aircraft nose will be higher when flying at a slower speed if it maintains straight and level flight!
However, a word of warning…
While it would be nice to have unlimited climb performance by increasing the angle of attack, aerodynamics doesn’t work like that.
Why?
Well, there are limits on how much you can increase the angle of attack before bad things happen.
Look at this graph showing the relationship between the angle of attack and the coefficient of lift.
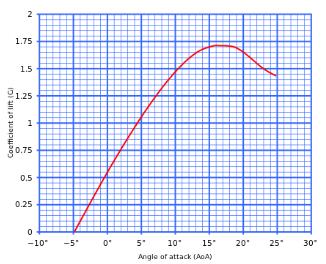
As the angle of attack increases, so does the lift coefficient. However, at a given point, the lift produced by the wing rapidly and markedly drops. This is because as a wing generates lift, it also generates drag.
There comes the point that, just after the ‘plateau’ of our curve, drag starts to overcome lift. As a result, the aircraft wing will stall. Or, to put it in really simple terms, stop producing lift. We call this the critical angle of attack.
You can see how drag increases in this graph
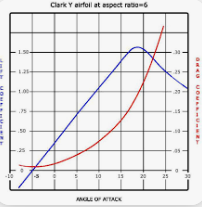
There are several factors that cause drag, and it is unavoidable. Once we start getting towards the top of the line we need more lift to counter the drag, which in turn creates more drag, and before you know it, the wing can’t keep up!
When an aircraft wing stops producing lift, there is only one place the aircraft is going…
Down!
If you want to read more about stalls, check out our guide here.
The Angle of Attack Vs. Pitch – Are They the Same Thing?
It is important to note that, while they can be related, aircraft pitch attitude is not the same as the angle of attack. The angle of attack is a relationship between the chord line and the oncoming airflow. Pitch attitude is the relationship between the aircraft nose and the horizon.
It might seem like we are dealing with semantics, but you must understand this concept.
Here’s why.
Let’s say you dive the aircraft down at a 45° angle towards the ground (from a safe altitude) before hauling back sharply on the control column.
Your pitch attitude is -45° during the early stages of the maneuver.
A low-pitch attitude means a low angle of attack, far away from our critical angle, right?
Nope!
Once the aircraft is stabilized in its dive, your pitch attitude is true -45°. However, that is regarding the earth’s horizon. The angle of attack, formed between the wing chord line and oncoming airflow, will be much closer to somewhere around 3°. To make life easy, let’s say the critical angle of attack (where the wing will stall) is 15°.
When you haul back on the control column, even though your pitch attitude is well below 15°, your angle of attack isn’t… meaning it won’t take much to exceed the critical angle. Many pilots have stalled in a dive.
In fact, you should remember this simple rule: –
- An airplane can stall at any altitude or airspeed if the critical angle of attack is reached.
Inverted, in a climb, a dive, wings level… It doesn’t matter. If the angle of attack exceeds a given value, you will stall the aircraft.
It is also possible to stall at high speed. Stalls tend to happen at slower speeds because the aircraft’s angle of attack is already high to ensure enough lift is produced to keep the aircraft flying. This leads to a high-nose attitude, which is why many incorrectly assume that high pitch and high angle of attack are always the same.
Why is Angle of Attack Important?
Knowing the theory behind AoA is vital if you are to be a safe pilot. There are quite a few areas where you will want to be acutely aware of your aircraft’s angle of attack. Here are the areas that you need to think about.
Stall Awareness
By now, it should be obvious. There are limits to how much you can increase α before you reach the critical angle of attack.
Remember, the aircraft will always stall at a set α value for a given configuration, regardless of attitude or speed.
Steep Turns
During the steep turns, there is an effect that makes reaching a high angle of attack much more likely.
As you ‘pull’ the aircraft around the turn, you create a gravitational effect. Ever noticed your arms and legs feel ‘heavy’ when making steep maneuvers? Essentially you are increasing the gravitational force on the aircraft.
And here’s the thing.
Let’s say you pull 2 G’s. During the maneuver, the aircraft (and everything in it) weighs twice as much. This means the wing has to work doubly hard to produce enough lift to maintain level flight. To counter the effect of this wing loading, the pilot has to pull on the stick.
You’ve guessed it…
Pulling on the stick increases alpha… Bringing you closer to the critical angle.
Slow Flight
Remember our equation above?
We saw that the lift produced is the interaction between the angle of attack, airspeed, and wing shape. If one of the trio is reduced, the other two must up their game to ensure the lift remains constant.
If we reduce the speed, we must increase the angle of attack to compensate.
Again, by increasing the angle of attack, we come closer to the critical angle.
Aircraft Weight
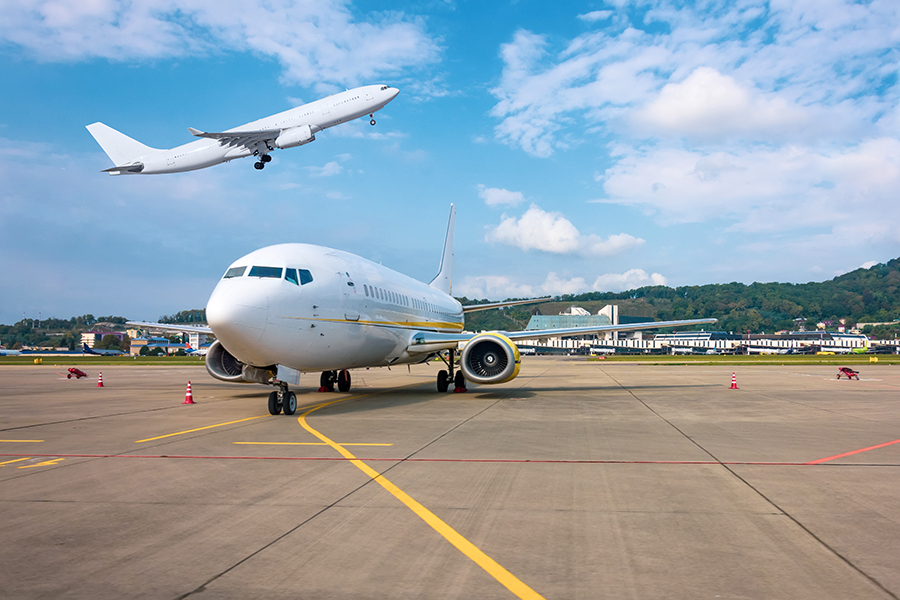
For an aircraft to maintain a level altitude, the lift produced must equal the aircraft’s weight.
If we increase the weight, then we also must increase the lift.
Make sense so far?
There are only two ways to increase the lift (and again, it relates to our equation above)…
Either we fly faster, or we increase the angle of attack.
Increasing speed isn’t always possible, and you’ll tend to find that at higher weights, the aircraft pitch attitude is higher. A higher pitch attitude generally means a higher angle of attack in level flight.
Stretching the Glide and Exceeding Critical AoA
While engine failures are exceptionally rare, they do happen. Often pilots exceed the critical angle of attack while trying to ‘stretch the glide’ and reach a landing site.
It is imperative that if you are gliding an aircraft, you don’t pull back to try and extend the aircraft’s engine out of range. Maintain your best glide speed, representing the optimum between lift and drag.
What Factors Affect Angle of Attack?
Several factors affect AoA. While airplane manufacturers suggest when the critical angle might be reached, it can vary daily, even with the same aircraft. Here’s a quick list of factors that may combine to affect the angle of attack.
Wing Size and Shape
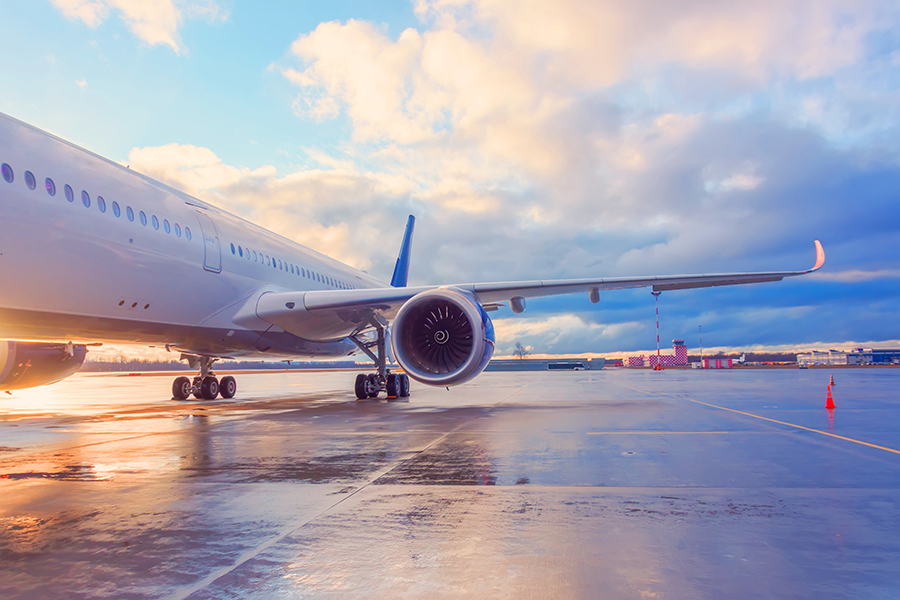
As a general rule, the larger the wing, the more lift it produces.
However, size isn’t everything.
The wing shape is just as important regarding the angle of attack.
Different wings offer a different lift-to-drag ratio. Some are designed to be benign in handling, whereas others (such as fighter aircraft wings) are designed to be inherently unstable.
Ice
There is one situation where nobody can guess what the critical angle of attack will be.
We are talking about icing.
Ice causes problems for two reasons when it comes to the angle of attack.
First, ice changes the shape of the wing and severely affects aerodynamics.
Second, even a small layer of ice can weigh a substantial amount.
It’s pretty bad.
More weight and an ‘experimental’ wing mean that the angle of attack is severely and unpredictably altered.
Wing Contamination
While not quite as severe as ice, other contaminants can affect the angle of attack. Clean wings are the most efficient. Dust, bird droppings, or general dirt all reduce this efficiency. This can lead to a change in the critical angle.
Load Factor
Load factor, while related to aircraft weight, is not the same thing.
Load factor is the apparent change to weight caused by ‘pulling G’.
And it can get a little dangerous when it comes to AoA.
Here’s why.
The stall speed (used by airplane manufacturers to give you a good idea of when you are reaching critical alpha) increases at the square root of the load factor.
Let’s say your stall speed was 60 knots. When pulling two G’s, you’ll find this increase by about 1.4!
60 X 1.4 = a new stall speed of 84 knots! Meaning the critical alpha is reached sooner!
Aircraft Speed
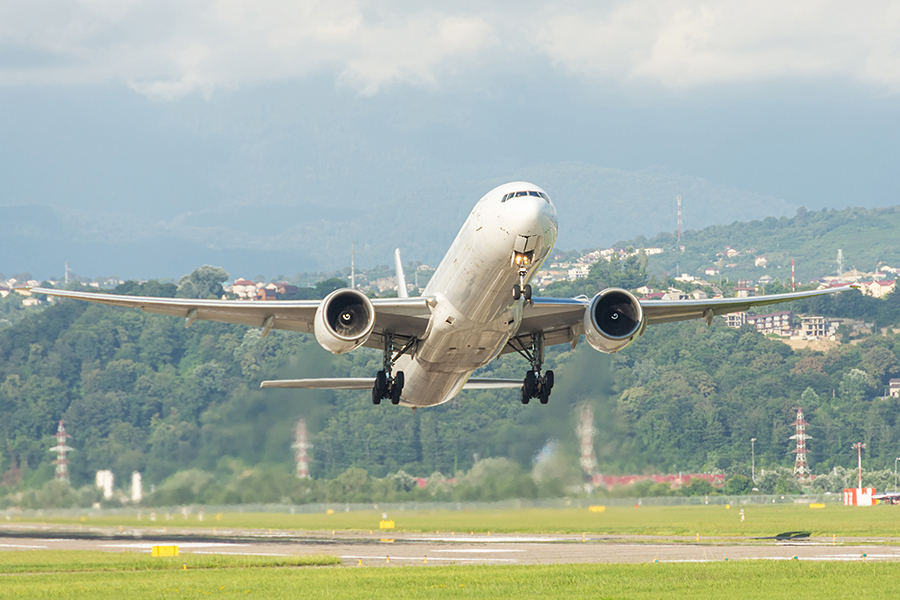
Remember that critical α can occur at any speed and configuration. However, there are circumstances where reaching the stall angle of attack is much more likely.
Most pilots come closest to the stall during low-speed phases of flight, such as during the landing or turning in the circuit. Because there is less airflow over the wing, there is less lift. This is compensated for by pulling back on the stick.
We know where this leads…
A slower flight requires a higher AoA to maintain the level. This puts you much closer to the critical angle.
Center of Gravity
One area to be hyper-aware of when it comes to AoA is your aircraft’s center of gravity.
If you want to learn about aircraft weight and balance, here’s a good guide.
In particular, you need to keep an eye on a forward center of gravity.
The aircraft’s tailplane has to apply more downforce to keep the aircraft in the correct position. More downforce means the wing has to work a little harder to produce the required lift.
Based on what we have learned, how do we normally achieve this?
By flying a higher angle of attack, of course!
Control Inputs
Remember, the AoA is a relationship between the chord line and the oncoming airflow. If you make a harsh control input, like, let’s say, a big pull, what will that do to the chord line in relation to the airflow?
It will cause an increase in the angle of attack.
Smooth control inputs mean that the difference between the oncoming airflow and chord line stays smaller. Large control inputs cause a bigger immediate difference.
High Lift Devices
As you’ll have seen above, we interchange the angle of attack, airspeed, or wing shape to produce a given amount of lift.
We can’t change the wing shape, can we?
Oh yes, we can!
Most aircraft have devices, such as slats and flaps, that allow us to temporarily alter the shape of the wing and change the angle of attack. As flaps lower, they change the position of the chord line as the trailing edge moves. This means that the wing gets bigger and also has a higher angle of attack, even if the speed stays the same!
More angle of attack and a bigger wing means more lift! Nice!
Final Thoughts
While the angle of attack, or alpha if you prefer, is simply the angular difference between the chord line and airflow, there is actually a lot to think about. Understanding AoA is vital for safe aviating. If you want to learn more about aviation, you are in the right place. Check out our free pilot course for other great lessons.