Drag – the enemy of flight (and the evil cousin of weight).
You likely understand the concept of drag in general, but there are two types of drag that affect an aircraft in flight:
- Induced Drag
- Parasite Drag
Parasite drag is fairly easy to understand. Induced drag, on the other hand, is more complex.
In this article, we’ll dive into what induced drag is, where it comes from, how it differs from parasite drag, and how we can avoid it (or use it to our advantage).
What Creates Induced Drag?
Induced drag is a consequence of producing lift. It is a direct result of wingtip vortices created by the difference in pressure between the top and bottom of the wing.
Let’s explain.
Imagine your aircraft’s wing. As air flows over the wing, it generates lift. The air pressure above the wing is lower than the air pressure below the wing. This difference in pressure creates an upward force we call lift.
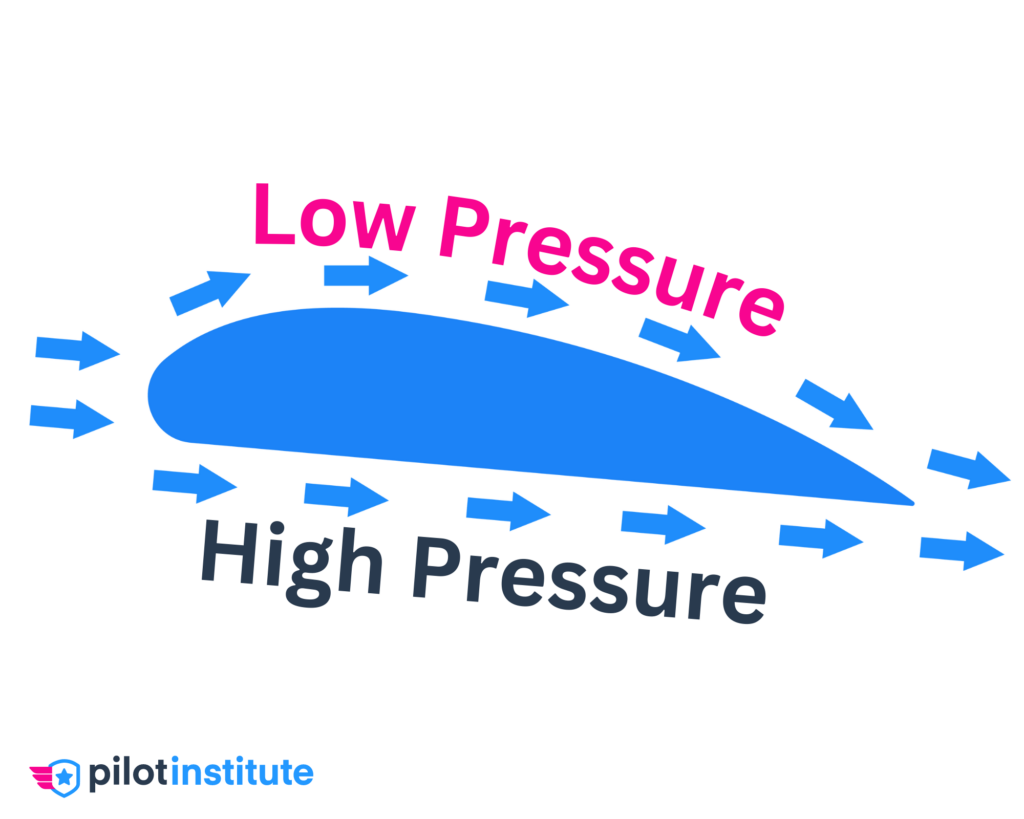
But lift comes at a price.
Air flows from high to low pressure. This means that the high-pressure air on the bottom of the wing desperately wants to go to the low-pressure side on top of the wing.
And where is the easiest place for the high-pressure to join its low-pressure friend? The wingtips!
This results in swirling air masses known as wingtip vortices. Think of them as mini tornadoes spiraling off the tips of your wings.
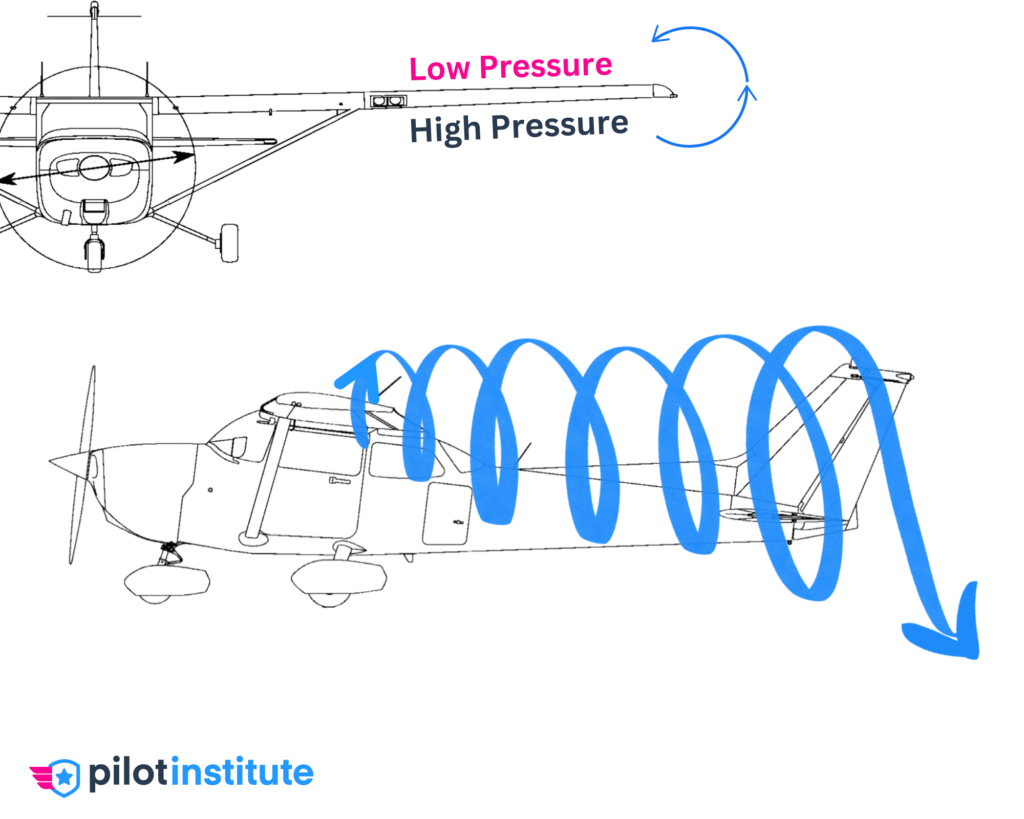
Now, here’s where induced drag steps in. The energy spent creating these vortices is energy that doesn’t contribute to forward movement, leading to drag on the aircraft – induced drag.
The stronger the vortices, the higher the induced drag.
It’s important to understand that the creation of the wingtip vortices causes induced drag, not necessarily the wingtip vortices themselves. But the two are obviously related – reducing wingtip vortices requires reducing the creation of wingtip vortices.
So, wingtip vortices are more than just mesmerizing spirals of air trailing behind an aircraft.
They’re a visible manifestation of induced drag.
Just as you can’t have a shadow without light, you can’t have a lift without drag.
Let’s take a look at what influences these wingtip vortices (and induced drag).
Induced Drag and Angle of Attack
The angle of attack of an aircraft is the angle between the oncoming air (known as “relative wind”) and a reference line on the wing called the chord line. The angle of attack is important because it significantly influences the amount of lift generated by the wing.
At low angles of attack, where the wing is more aligned with the airflow, induced drag is relatively low. This is because the pressure differential between the top and bottom of the wing isn’t as pronounced, resulting in weaker wingtip vortices.
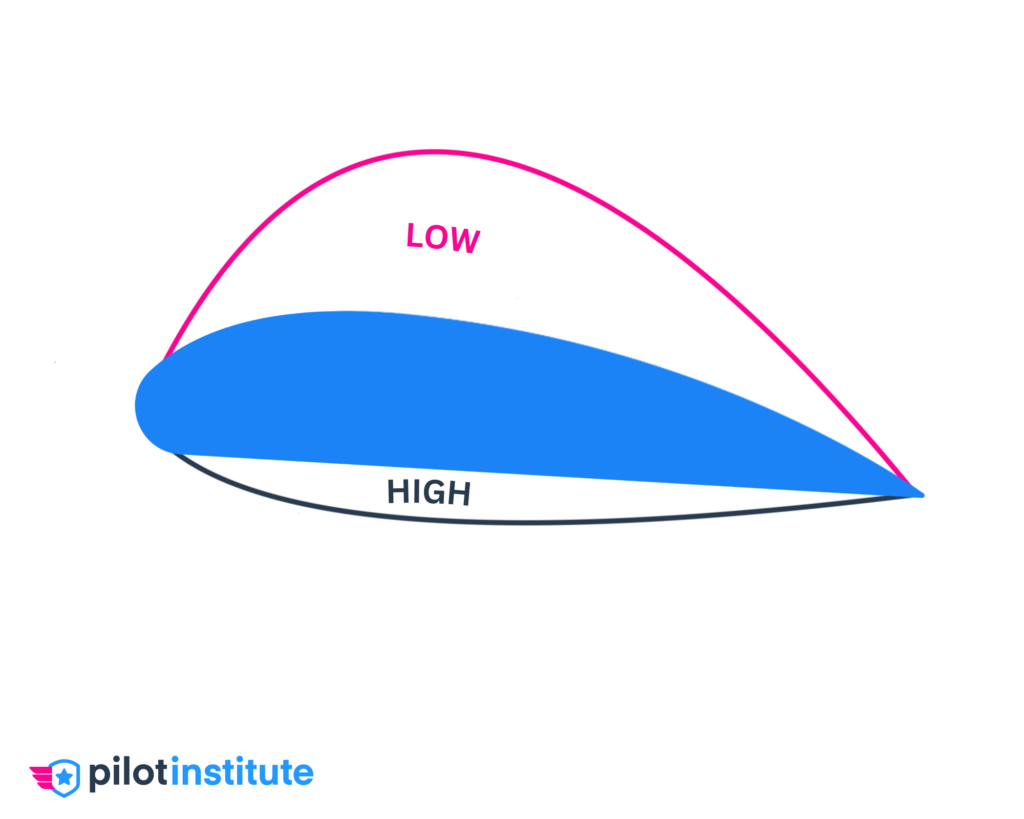
However, as the angle of attack increases, the wing generates more lift, and the pressure difference between the top and bottom of the wing becomes greater. This leads to stronger wingtip vortices and, consequently, more induced drag.
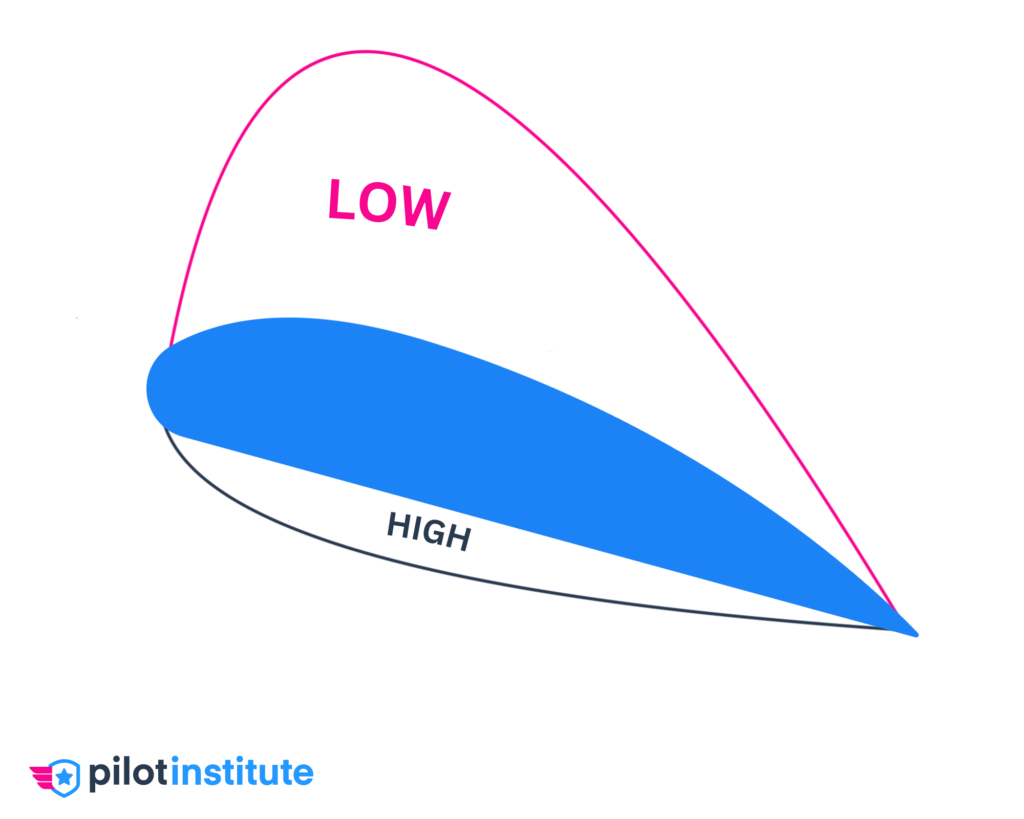
Beyond a certain critical angle of attack, the airflow begins to separate from the upper surface of the wing, causing a rapid loss of lift, known as a stall.
So, in summary, at low airspeeds or high angles of attack (like during takeoff and landing), induced drag is higher due to the increased strength of the wingtip vortices.
Conversely, at high airspeeds or low angles of attack (like during cruise), induced drag is lower.
That’s why when an aircraft is heavy, in the clean configuration (no flaps), and slow, it produces the largest wingtip vortices – because the angle of attack is high.
Okay, great. So as long as we’re flying fast, we won’t have to worry about drag, right? Unfortunately not, because of the second type of drag: Parasite drag.
Parasite vs. Induced Drag
Parasite drag is the ‘uninvited guest’ in the world of aviation. Unlike induced drag, which decreases as your speed increases, parasite drag does the exact opposite. It grows with speed, becoming more of a nuisance as you fly faster.
Parasite drag is broken into three types:
- Form Drag: Arises from the aircraft’s shape disrupting the air it moves through
- Skin Friction: The result of air’s viscosity, causing resistance as it flows over the aircraft’s surface.
- Interference Drag: Stems from the turbulent airflow at the junctions of the aircraft’s parts, like where the wings meet the fuselage.
Parasite drag is all about resistance.
In contrast, induced drag, which is related to the production of lift and the creation of wingtip vortices, decreases as your speed increases. This means that when you’re flying slowly, induced drag dominates. As you gain speed, induced drag lessens, and parasite drag starts to take over.
In essence, these two types of drag have an inverse relationship with speed, creating a tug-of-war that pilots must manage for efficient flight.
“Total Drag” is the sum of parasite and induced drag.
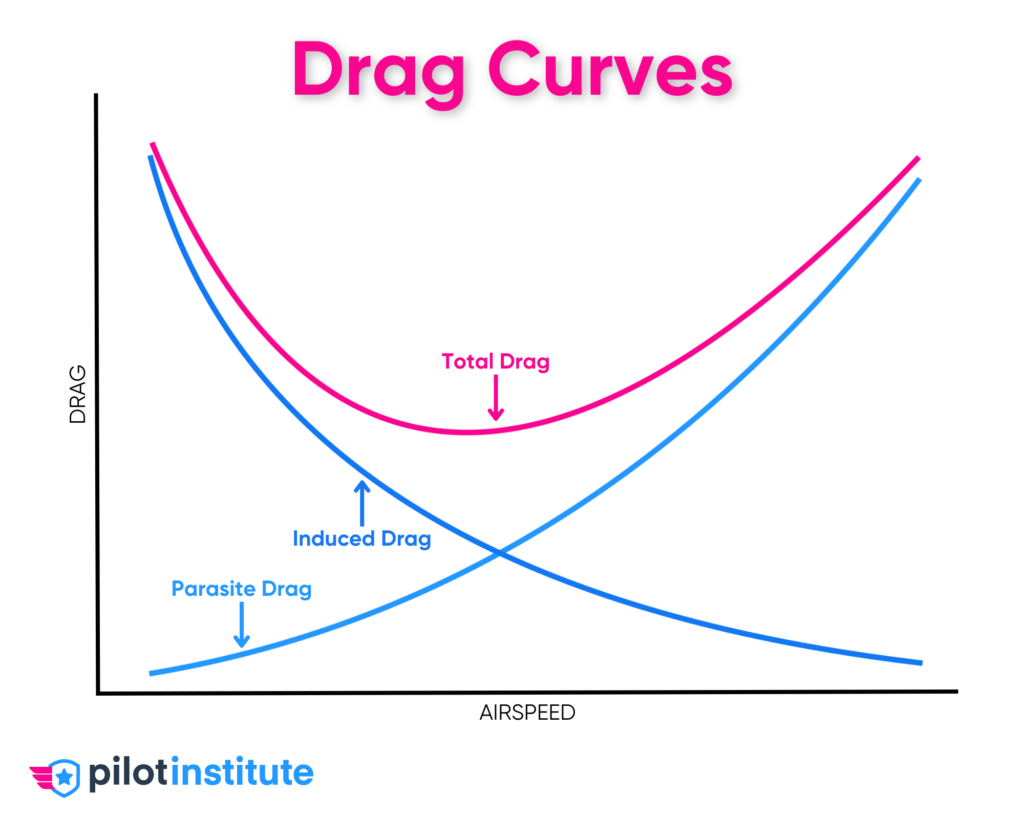
Wake Turbulence and Induced Drag
Wake turbulence and induced drag are closely linked, and understanding their relationship can be crucial for safety in the skies.
Wake turbulence is the disturbed air left behind an aircraft as it flies. Think of it as the ‘footprint’ your aircraft leaves in the sky. Now, remember those wingtip vortices we mentioned when we discussed induced drag? They are the primary contributors to wake turbulence.
Wake turbulence poses a particular risk for smaller aircraft flying behind larger ones because the vortices from the larger aircraft can cause severe upsets. That’s why air traffic control ensures a safe separation distance between larger and smaller aircraft during takeoff, landing, and flight.
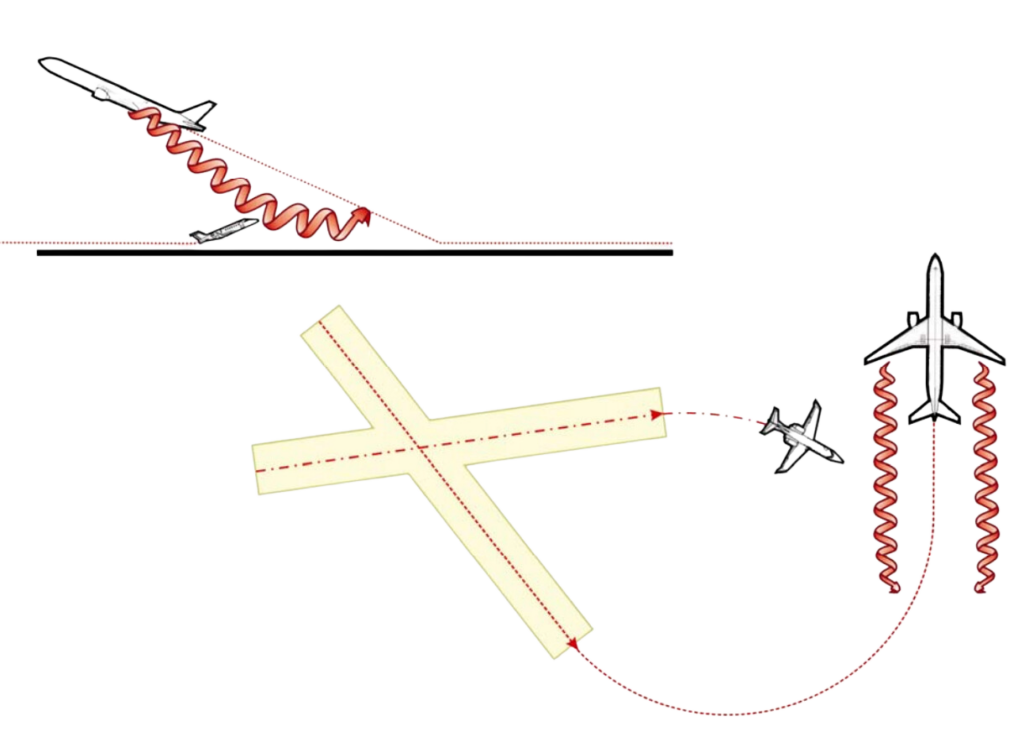
Ground Effect and Induced Drag
Ground effect is a term you’ll often hear in the context of takeoffs and landings. But did you know that it’s also related to induced drag? In fact, understanding the ground effect can be quite beneficial for managing your aircraft’s performance.
The ground effect occurs when an aircraft is flying very close to the ground, typically at an altitude less than the wingspan’s length. In this region, the wing’s proximity to the ground interferes with the formation of the wingtip vortices, which, as we’ve learned, are the major contributors to induced drag.
Here’s how it works: when you’re flying close to the ground, the surface restricts the vertical movement of the air, effectively ‘squashing’ the vortices. This reduces their strength, which in turn reduces the induced drag.
So, what does this mean for you as a pilot? Well, when you’re taking off, the ground effect can help your aircraft ‘float’ a little before it reaches the optimal climb speed. Similarly, during landing, it can provide a cushioning effect, making your touchdown smoother.
Reducing Induced Drag
Aircraft designers have a toolbox full of clever tricks to reduce induced drag. They know it’s a factor in every flight, so they engineer ways to mitigate its effects. Let’s explore some of the design features they employ to keep induced drag in check.
Winglets
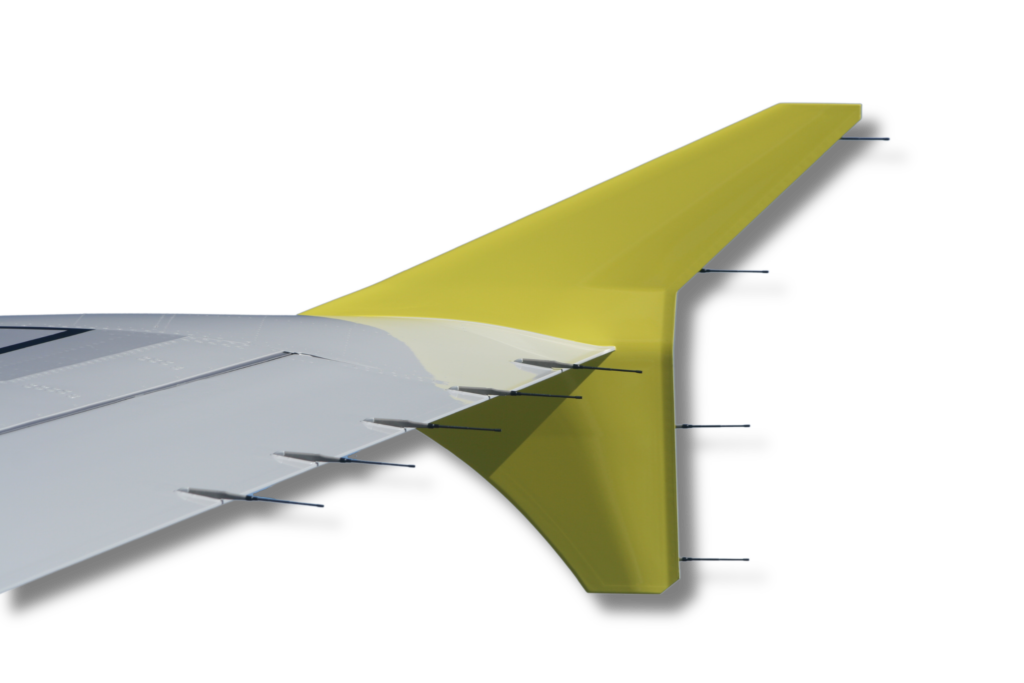
One of the most visible features is a wingtip device known as a winglet or sharklet, often found on modern airliners.
These upward or downward extensions at the wingtips disrupt the formation of wingtip vortices, reducing induced drag. They’re like a physical barrier that keeps high-pressure air from rolling up and over the wingtip into the low-pressure area above.
Aspect Ratio
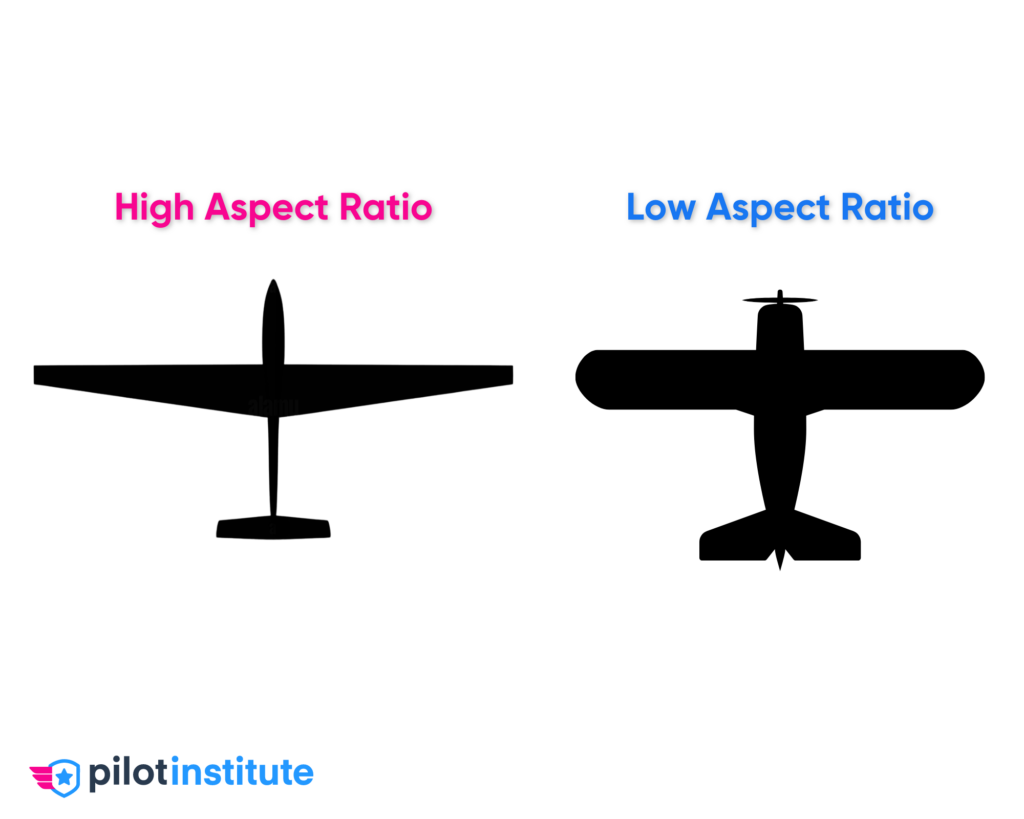
Another approach is increasing the aspect ratio of the wing – the ratio of the wing’s length to its width. Wings with a high aspect ratio (long and narrow) are better at minimizing induced drag than wings with a low aspect ratio (short and wide). This is because longer wings have less pronounced wingtip vortices.
Wing Shape
Designers also consider the wing’s shape, specifically its ‘airfoil’ profile. Certain airfoil shapes can produce lift more efficiently, reducing the strength of the vortices and, thus, the induced drag.
Washout
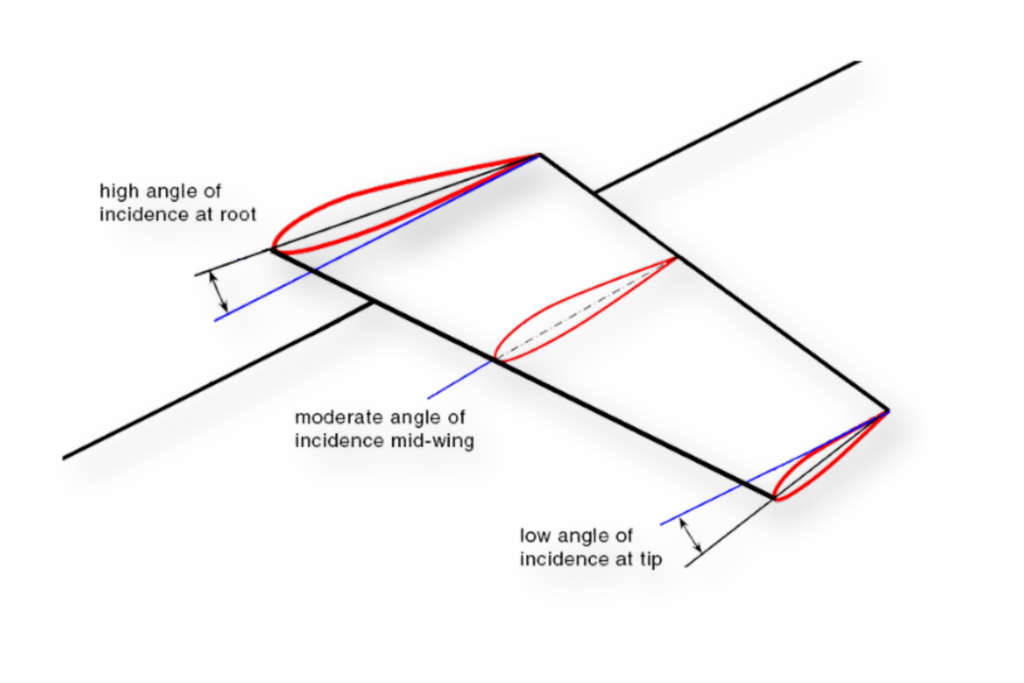
Finally, some aircraft utilize a feature called ‘washout.’ This is a twist in the wing that causes the wingtips to have a lower angle of attack than the wing roots. Washout can help reduce the intensity of wingtip vortices and the resultant induced drag.
Summary
Induced drag, an essential part of the flight puzzle, may seem intimidating at first. However, once you understand the concepts of lift, pressure differences, wingtip vortices, and angle of attack, it begins to make a lot more sense.
Remember, induced drag is an unavoidable consequence of producing lift. It’s the result of high-pressure air escaping to the low-pressure area above the wing, creating spiraling wingtip vortices. These vortices are essentially the visual representation of induced drag.
Induced drag is influenced by your angle of attack and your speed. When you’re flying slowly or at high angles of attack, induced drag dominates. As you speed up, induced drag decreases, but be aware, this is where parasite drag steps in.
It’s a game of balance between these two types of drag, and finding that sweet spot of efficient flight requires understanding and managing both.
Ground effect and wake turbulence are two phenomena closely related to induced drag. Understanding their relationship to induced drag is crucial for your safety and performance during takeoffs and landings.
Finally, aircraft designers go to great lengths to reduce induced drag. They employ clever tricks like winglets, high aspect ratio wings, specific airfoil shapes, and washout.
So, the next time you see those swirling vortices off an airplane’s wingtip, remember, you’re seeing a visual representation of a complex, yet fundamental aspect of flight – induced drag.